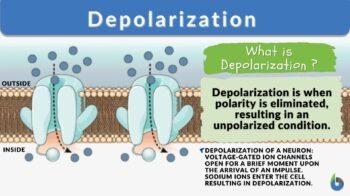
Depolarization
n., plural: depolarizations
[diːˌpəʊ.ləɹaɪˈzeɪ.ʃən]
Definition: The deprivation or the process of neutralization of polarity, as that in nerve cells
Table of Contents
Depolarization is the removal of polarity by a process or action. It might also be used to describe how such activity leads to an unpolarized situation as the outcome. Depolarization takes place in a live cell, such as a nerve cell, in biology. When a nerve cell experiences an electrical shift, depolarization happens.
Magnetic charges are found among many walks of nature. It is one of the ways that chemistry and biology work together in order to carry out processes within the body. Typically, cells can be depolarized and repolarized. Repolarization is a step of an action potential known as repolarization that occurs when the cell experiences a drop in voltage due to the outflow of potassium (K+) ions along its electrochemical gradient of it.
So if this is repolarization, what does depolarization mean? What happens in the depolarization of an organism’s body part such as a neuron or cell depolarization? To define depolarization, one must consider the depolarization – biology definition. This definition can often be unanimous with depolarization – psychology as this is often used for neuron depolarization.
Depolarization Definition
Depolarization is when a change occurs inside a cell that causes the distribution of electric charges to alter, leaving the cell with a less negative charge than the outside. Numerous cell functions, cell-cell communication, and the general physiology of an organism all depend on depolarization. The depolarization phase is shown in the depolarization diagram below (Figure 1).
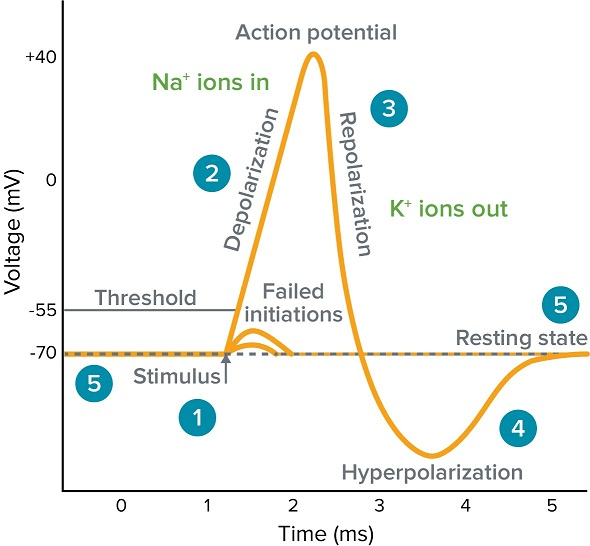
What causes depolarization? It is when the voltage-gated sodium channels open. This causes the positively charged sodium ions to flood into a neuron. This causes depolarization. During depolarization, the cell’s normally negative internal charge momentarily changes to a positive one (less negative). Several activities, including action potential, result in this membrane potential transition from a negative to a more positive state.
What is the depolarization of a neuron? Positively charged sodium ions (Na+), commonly known as the rising phase, enter a neuron through open voltage-gated sodium channels to generate depolarization. In fact, the membrane potential changes polarity as more sodium rushes in.
What is depolarization of the heart? The process of depolarizing the heart involves the sequential, orderly flow of electrical current through the heart muscle, converting each cell from its resting polarized state to a depolarized one until the entire heart has undergone depolarization.
General kinds of depolarization in the body include:
- Muscle depolarization
- Neuron depolarization
- Membrane depolarization
- Cardiac depolarization
- Mitochondrial depolarization
- Cardiomyocyte depolarization
Watch this vid about biological depolarization:
Biology definition:
Depolarization is the process or the act by which polarity is eliminated. It may also refer to the result of such action so that the result is an unpolarized condition. In physiology, depolarization occurs in a living cell (e.g. nerve cells).
Example:
- Depolarization in a nerve cell occurs when the cell undergoes an electrical shift. Most cells are negatively charged relative to their surroundings. This negative internal charge of the cell shifts to a positive through depolarization, which occurs for only a brief period of time. This type of depolarization is essential because it allows the transmission of electrical signals (impulses) within the cell, and in certain instances, from one cell to another. Depolarization, therefore, is a mechanism employed by cells to carry on certain physiological functions and also to communicate with other cells.
Etymology: Latin dē (from, away from, out of) + polarization
Variant: depolarisation
Compare: polarization
Physiology
The inherent electrical nature of most cells plays a key role in the depolarization process. A cell retains what is referred to as a resting potential when it is at rest. The interior of the cell has a negative charge relative to the exterior due to the resting potential created by almost all cells. Ions, tiny positively and negatively charged relatives that are carried across the plasma membrane of the cell to maintain this electrical imbalance.
Several distinct transmembrane proteins, including ion channels, sodium-potassium pumps, and voltage-gated ion channels, which act as channels for ions both into and out of the cell, are embedded in the plasma membrane of the cell and help to transport ions across the plasma membrane.
-
Resting potential
Before a cell may be depolarized, the resting potential must be established. Although a cell can build a resting potential through a variety of processes, most cells similarly generate resting potentials.
Negative resting potential is produced inside the cell via ion channels, ion pumps, and voltage-gated ion channels. However, the process of producing the resting potential inside the cell simultaneously produces a depolarizing environment outside the cell.
The sodium-potassium pump plays a key role in creating ideal depolarization conditions on the inside and outside of the cell. The resting potential of the cell is established by pumping three positively charged sodium ions (3 Na+) out of the cell for every two positively charged potassium ions (2 K+) pumped in, and an unfavorable concentration gradient is produced by raising the concentration of sodium outside the cell and decreasing the concentration of potassium inside the cell.
The resting potential that is generated will keep the voltage-gated ion channels in the cell membrane closed. As a result, the ions pumped across the membrane will be prevented from diffusing back to the area of relatively lower concentration. Consequently, there will be a relatively greater concentration of potassium inside the cell while more sodium outside the cell.
Additionally, the majority of cells contain internal components (with a negative charge), which add up to generate a negative inner charge despite the enormous quantity of positively-charged potassium ions.
-
Depolarization
A cell has the ability to undergo depolarization once it has reached its resting potential. The membrane potential quickly changes from negative to positive during depolarization. Several actions must take place along the cell’s plasma membrane for this quick shift to happen within the cell.
The voltage-gated ion channels (voltage-gated sodium channels and voltage-gated calcium channels) that had been closed while the cell was at resting potential open in response to an initial shift in membrane voltage, and the sodium-potassium pump continues to function.
The membrane potential changes from negative to positive when the sodium ions rush back into the cell, adding a positive charge and changing the interior of the cell’s charge. Depolarization of the cell is complete when the interior becomes a more positive value, at which point the channels close once more.
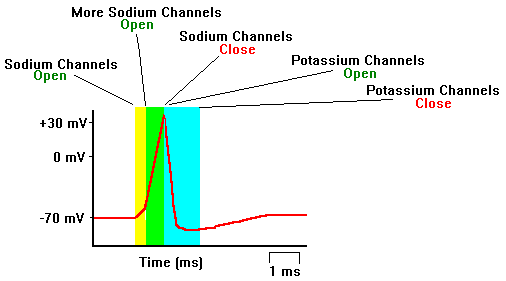
-
Repolarization
After being depolarized, a cell’s inherent charge changes one last time. The voltage-gated sodium ion channels that were open throughout the depolarization process in the cell shut once more after depolarization.
The potassium channels now open as a result of the cell’s increased positive charge. K+ ions start to descend the electrochemical gradient (in favor of the concentration gradient and the newly established electrical gradient). The potential inside the cell diminishes when potassium leaves it and returns to its resting potential. Throughout this procedure, the sodium-potassium pump is constantly in operation.
Hyperpolarization
The cell’s potential overshoots as a result of the repolarization process. The amount of potassium ions exiting the axon exceeds the resting potential, making the new cell potential more negative than the resting potential. The sodium-potassium ion pump is active, and all voltage-gated ion channels are closed, and this restores the resting potential.
Neurons
An overshoot in the cell’s potential results from the repolarization process. Potassium ions keep leaving the axon until they exceed the resting potential and the new cell potential turns more negative than the resting potential. The sodium-potassium ion pump is activated, all voltage-gated ion channels close, and the resting potential is finally restored.
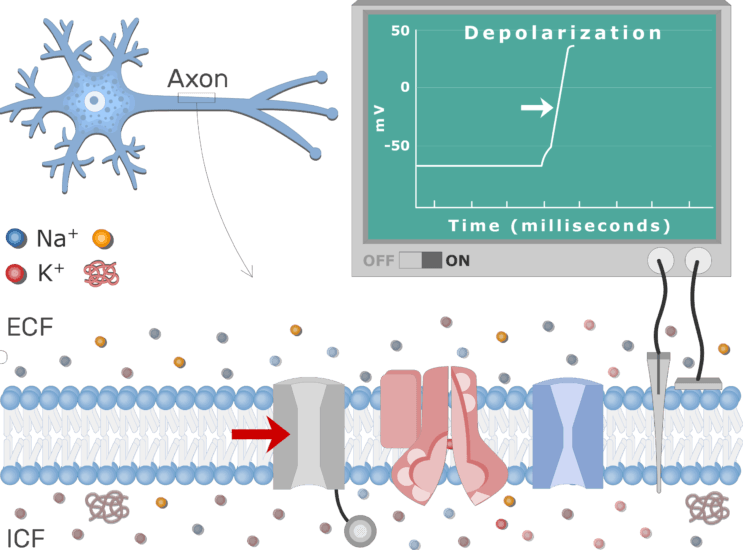
-
Response to stimulus
Physical, electrical, and chemical stimuli to neurons can either inhibit or excite the neuron that is being activated. A neuron’s dendrite receives an inhibitory signal, which leads to the neuron’s hyperpolarization. A further drop in voltage below the resting potential occurs in the cell as a result of the hyperpolarization that occurs after an inhibitory stimulation.
An inhibitory stimulus causes a larger negative charge in a hyperpolarized neuron, which must be overcome for depolarization to take place. On the other hand, excitation events raise the neuron’s voltage, making it simpler to depolarize than the identical neuron in the resting state. The stimulus passes from the dendrites of a neuron to the cell body for integration, whether it is excitatory or inhibitory.
-
Integration of stimuli
Before the nerve may react, the numerous impulses must be integrated after they have reached the cell body. The neural response is calculated by adding the stimuli that have converged at the axon hillock after traveling down the dendrites. The axon depolarizes from the axon hillock down if the total voltage of the stimuli exceeds a certain value, known as the threshold potential or threshold value.
-
Response
A depolarization action potential is a burst of depolarization that moves from the axon hillock to the axon terminal. Action potentials go to the axon terminal, where they cause the cell to release neurotransmitters. Other cells, such as muscle cells or other neurons, are stimulated by the neurotransmitters that are produced by the axon.
The resting membrane potential of the axon must be restored after an action potential has passed through the axon of a neuron in order for another action potential to proceed. The neuron cannot send another action potential during this time, which is referred to as the recovery period of the neuron.
-
Rod cells of the eye
The interaction between rod cells and the accompanying neurons in the eye is a good example of the significance and adaptability of depolarization inside cells. Rod cells get depolarized in the absence of light. Due to the increased voltage of the rod cell in the depolarized state, ion channels in the rod cells are able to sustain this depolarization.
The ion channels enable unfettered entry of calcium and sodium into the cell, maintaining the depolarized condition. Neurotransmitters are continuously released by depolarized rod cells, stimulating the rod cell-related neurons. When rod cells are exposed to light, the channels that had previously made it easier for sodium and calcium to enter the rod cell are closed, breaking the cycle.
The rod cells generate fewer neurotransmitters when these channels close, which the brain interprets as more light. Depolarization thereby inhibits a signal from reaching the brain in the case of rod cells and the neurons that are linked with them rather than encouraging the transmission of the signal.
Vascular endothelium
Simple squamous epithelial cells form the thin layer of endothelium that lines the inside of blood and lymph vessels. Vascular endothelium, the endothelium that lines blood vessels, is susceptible to and must endure the stresses of blood flow and blood pressure from the cardiovascular system.
Endothelial cells need to have a structure that can withstand the forces of circulation while also keeping a certain degree of flexibility in the strength of their structure in order to endure these cardiovascular stresses. The whole performance of the cardiovascular system depends on this adaptability in the structural strength of the vascular endothelium.
The strength of the structure of endothelial cells in blood vessels may be changed to maintain the vascular tone of the blood vessels they line, avoid vascular stiffness, and even assist in controlling blood pressure in the cardiovascular system.
Depolarization, which modifies the structural strength of endothelial cells, enables them to do these feats. The network of fibers that provide endothelial cells with their structural support is altered during depolarization, which causes a noticeable reduction in the stiffness and structural strength of the cell.
In addition to maintaining the structural integrity of endothelial cells, vascular endothelium has to be depolarized in order to regulate vascular tone, avoid vascular stiffness, and regulate the flow of blood through the body.
Heart
Both atria first, followed by both ventricles, are where depolarization occurs in the heart’s four chambers.
- The right and left atria contract as a result of depolarization brought on by the sinoatrial (SA) node, which is located on the wall of the right atrium. This contraction is shown by the P wave on an ECG.
- The atrioventricular (AV) node receives the depolarization wave from the SA node, and after a delay of around 100 ms to allow the atria to complete contracting, produces a contraction in both ventricles, as seen in the QRS wave. The atria also relax and re-polarize at the same moment.
- At the T wave, the ventricles are depolarized and relaxed.
Unless there is a cardiac issue, this procedure continues routinely.
Frequent ventricular ectopic impulses cause left ventricular (LV) dysfunction and systolic heart failure (HF) in a syndrome known as ventricular premature depolarization (VPD)-induced cardiomyopathy. A ventricular premature depolarization ICD-10 can be used to assist with this issue.
Watch this vid about cardiac action potential:
Depolarization Blockers
Depolarization blocking medicines are medications that activate the channels responsible for depolarization but do not let them shut, preventing repolarization. This results in prolonged depolarization. Suxamethonium and decamethonium, nicotinic agonists, are two examples.
Quick Facts!
Research on the giant squid axon — the largest known nerve cell in the animal kingdom– has taught researchers a lot about how depolarization in neurons functions.
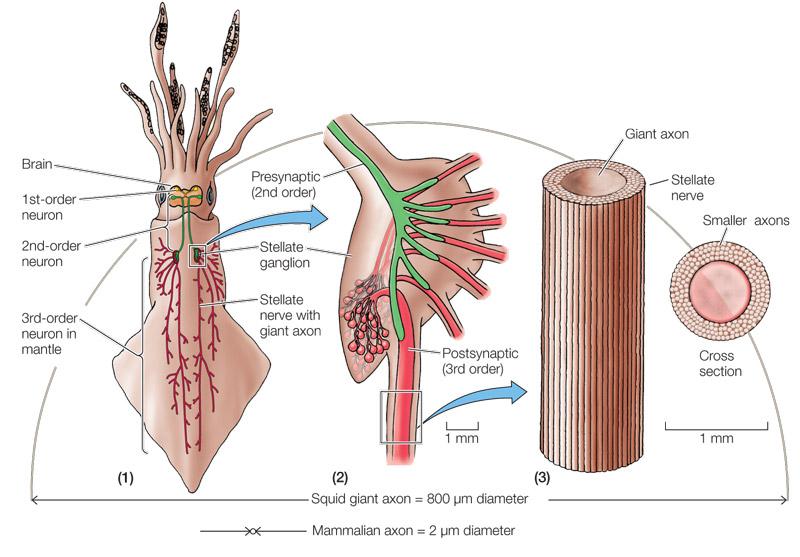
Answer the quiz below to check what you have learned so far about depolarization.
References
- 10.5E: The Action Potential and Propagation. (2018a, July 20). Medicine LibreTexts. https://med.libretexts.org/Bookshelves/Anatomy_and_Physiology/Book%3A_Anatomy_and_Physiology_(Boundless)/10%3A_Overview_of_the_Nervous_System/10.5%3A_Neurophysiology/10.5E%3A_The_Action_Potential_and_Propagation
- 10.5E: The Action Potential and Propagation. (2018b, July 20). Medicine LibreTexts. https://med.libretexts.org/Bookshelves/Anatomy_and_Physiology/Book%3A_Anatomy_and_Physiology_(Boundless)/10%3A_Overview_of_the_Nervous_System/10.5%3A_Neurophysiology/10.5E%3A_The_Action_Potential_and_Propagation
- General Review. (n.d.). Retrieved August 6, 2022, from http://www.unm.edu/~lkravitz/UNM%20Pages/generalrev.html
- Lodish, H. F. (2000). Molecular cell biology. New York : W.H. Freeman. http://archive.org/details/molecularcellbio00lodi
- Molecular cell biology: Lodish, Harvey F : Free Download, Borrow, and Streaming: Internet Archive. (n.d.). Retrieved August 6, 2022, from https://archive.org/details/molecularcellbio00lodi/page/1021/mode/2up
©BiologyOnline.com. Content provided and moderated by Biology Online Editors.