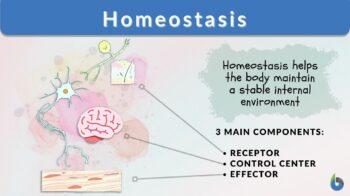
Homeostasis
n., plural: homeostases
[ˌhoʊ.mi.oʊˈsteɪ.sɪs]
Definition: the ability or tendency to adjust to achieve a stable internal environment
Table of Contents
Homeostasis is the tendency not to stray from the range of favorable or ideal internal conditions. Such conditions must be kept the same constantly. Maintaining a stable internal condition is crucial to any form of living thing. Different physiological strategies are employed to maintain the proper functioning of a system in spite of the external environment’s dynamicity. In fact, this capability is one of the hallmarks of being alive.
Instead of doing nothing, it acts upon and resists the effect of external factors so as not to deviate from the state of equilibrium, stability, or balance that it favors. In humans, homeostasis is maintained through regulatory mechanisms, each comprised of three general components: a receptor, a control center, and an effector.
The homeostatic mechanism may be in the form of a loop, which can either be positive or negative. As a feedback control system, the homeostatic process can therefore be conceptualized as a closed-loop structure, meaning the output of the system is fed back into the system as input, thereby regulating future action. Positive feedback leads to more stimulation or acceleration of the process whereas negative feedback leads to the inhibition of the (source of) stimulus or to the deceleration of the process.
Examples of positive feedback are labor contractions at childbirth, blood clotting, and action potential generation. Examples of negative feedback systems are thermoregulation, blood glucose regulation, baroreflex in blood pressure, calcium homeostasis, potassium homeostasis, and osmoregulation.
Homeostasis Definition
Homeostasis definition in biology is the ability or tendency of the body or a cell to seek and maintain a condition of equilibrium – a stable internal environment — as it deals with external changes. It makes use of feedback controls and other regulatory mechanisms or dynamic processes in order to maintain a constant internal environment. It can be construed as a skill of a living organism in its effort to stay within the optimal range despite fluctuating environmental conditions. Thus, in the biological context, the word homeostasis entails multifarious physiological control mechanisms in order to sustain and stabilize the functional, normal status of an organism.
Etymology: The term homeostasis comes from the Ancient Greek ὅμοιος (hómoios, meaning “similar”), from στημι (hístēmi, “standing still”) and stasis, from στάσις (stásis, meaning “standing”). The concept of homeostasis was first described in 1865 by Claude Bernard, a French physiologist. However, the term was coined later in 1962 by the American physiologist Walter Bradford Cannon. Variant: homoeostasis.
Homeostatic Processes
An organism needs a system that effectively interconnects various biological processes and functions. The human body, for instance, has bodily organs made up of cells functioning in unison.
These organs, although distinct from one another, have to work alongside each other in order to sustain a set of internal conditions within the ideal range. There are various homeostatic processes and each of them works by regulating certain variables of the organism’s internal environment.
Homeostasis in the Human Body
The human body would not be able to function efficiently if there is a prolonged imbalance in the internal physical conditions and chemical composition. Just like any other living thing, the human body employs various homeostatic mechanisms to sustain its optimal functioning.
Variables such as body temperature, pH, sodium level, potassium level, calcium level, and blood sugar level have to be kept within the homeostatic range. The homeostatic range is defined as the allowable upper and lower limits for a particular variable. If beyond the range of homeostatic values, the body would soon fail to carry out its tasks and become dysfunctional. In order for the body to keep these variables within efficacious limits, various regulatory mechanisms are employed and each of them is comprised of three general components.
Components of Homeostasis
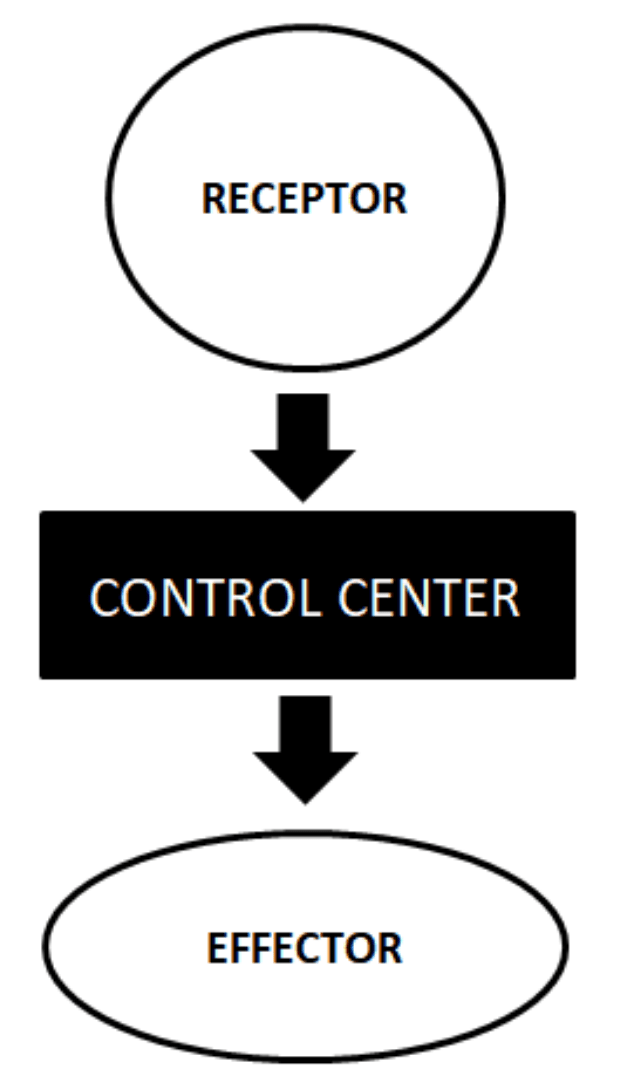
The components of homeostasis are: (1) a receptor, (2) a control center, and (3) an effector. The receptor, as the name implies, is that part of a homeostatic system that receives information regarding the status of the body. It monitors and perceives the changes in its environment, both internal and external. It is in the form of a sensory nerve terminal that receives the information (i.e. stimulus) and then responds by producing a nerve impulse according to the type, presence/absence, or extent of stimulation. Examples of receptors in the human body are as follows:
- Photoreceptors, i.e. receptors that react to light stimuli
- Olfactory receptor cells, i.e. receptors in the olfactory epithelium at the roof of the nose that react to odors or smell
- gustation receptors, i.e. receptors for taste
- Auditory receptor cells, i.e. receptors in the epithelium of the organ of Corti that react to sound stimuli
- Thermoreceptors, i.e. receptors in a sensory cell sensitive to changes in temperature
- Mechanoreceptors, i.e. receptors in the skin that reacts to various mechanical stimuli
- Interoceptors, i.e. receptors that respond to stimuli inside the body
- Nociceptors, i.e. receptors responsible for detecting or responding to pain
- Peripheral chemoreceptors, i.e. receptors that respond to chemical changes in the blood, e.g. oxygen concentration
The control centers pertain to the homeostatic component that processes impulses relayed by the receptors. Examples are the respiratory center and the renin-angiotensin system.
The effectors are the target of the homeostatic response that would bring about the reversion of conditions to the optimal or normal range. At the tissue or organ level, they are exemplified by the muscle or the gland. At the cellular level, they are the receptors of a nerve, including the nuclear receptors.
These three components work by first detecting and then responding to the information (i.e. stimulus) by the receptors of sensory cells. These cells respond to the detected change in the environment by relaying the information to the control center for processing, or directly to a particular target effector. Processing in the control center entails deliberation and determination of the appropriate response to the relayed stimuli. Then, it sends this message to the effectors. The effectors upon receiving the message would bring about the supposed response that would revert to the normal homeostatic range. At the cellular level, the activated nuclear receptors will act by upregulating (or by downregulating) the expression of certain gene(s). The protein produced from the gene expression would then exert its effect on the target organ.
Homeostatic Mechanisms
Homeostatic mechanisms that respond to a perturbation may be in the form of a looping mechanism (called feedback mechanism) that may be positive or negative. Positive feedback maintains the direction of the stimulus. It tends to accelerate or promote the effect of the stimulus.
Examples are labor contractions, blood clotting, and action potential generation. Negative feedback is a self-regulatory system and is employed in various biological systems. It reverses the direction of the stimulus and tends to inhibit the source of the stimulus or slow down the metabolic process. Examples include thermoregulation, blood glucose regulation, baroreflex in blood pressure, calcium homeostasis, potassium homeostasis, and osmoregulation.
Labor contractions
Labor contraction during childbirth is positive feedback since the initial contraction of the uterine muscle leads to further contractions. Rather than inhibiting the contraction, the body tends to produce more contractions. During labor, the posterior pituitary gland releases oxytocin which stimulates muscle contraction. At child delivery, oxytocin release is further augmented, intensifying muscle contractions until the neonate is pushed outside the birth canal.
Blood clotting
The formation of a blood clot in the presence of a tissue injury is an example of positive feedback. The conversion of blood from a liquid into a solid form entails a series of activations of clotting factors. As soon as one clotting factor is activated, the next clotting factor is activated, resulting in the formation of a fibrin clot. In this process, the direction of the stimulus is maintained.
Action potential generation
In neuron signaling, positive feedback is demonstrated during membrane depolarization. As nerve impulse is relayed along the axon of the neuron, voltage-gated sodium channels open in a series down the axon. The first set of voltage-gated sodium channels open, resulting in the influx of sodium ions. This, in turn, causes the depolarization of the surrounding area, which means the next set of voltage-gated sodium channels will open.
Thermoregulation
Thermoregulation is an example of negative feedback. It refers to the homeostatic regulation of body temperature. The human body tends to maintain an internal temperature of about 98.6 degrees Fahrenheit (98.6 ˚F, equivalent to 37 ˚C), also referred to as the set point. The core temperature is regulated chiefly by the nervous system, particularly the anterior hypothalamus and the preoptic area of the brain of the central nervous system.
When the ambient temperature is less than the skin temperature, heat loss occurs. This means that in colder surroundings (e.g. during the cold winter season) the body loses heat mainly from the hands and the feet. As a result, the core temperature falls. This is picked up by the thermoregulatory center of the brain as an unstable internal environment and initiates control mechanisms that aim to return the core temperature to the set point. One of the homeostatic mechanisms is by shivering to generate heat.
The thermoregulatory center in the brain sends signals to the muscles to shiver. Since the body remains still while shivering less heat will be dissipated to the environment.
On the other way around, when the ambient temperature is higher than the skin temperature, the body gains heat and consequently, the core temperature rises. This occurs during the hot summer days. The thermoregulatory control center in the brain responds, for example by stimulating the eccrine sweat glands to secrete sweat to cool the body off (by evaporative cooling).
Thermoregulation is an important homeostatic mechanism not just in humans but also in mammals.
Mammals maintain a constant body temperature that makes them characteristically warm-blooded. The body maintains an optimal core temperature through internal physiological regulation by a bodily system comprised of thermoreceptors in the hypothalamus, the brain, the spinal cord, the internal organs, and the great veins.1 Another way is allostasis, which is a behavioral form of homeostatic regulation.
For instance, during hot weather, they tend to seek shady, cooler places, and/or they do not move around a lot. During the cold season, they look for warm spots and they tend to increase their activity. Some species, such as birds, huddle or nestle together for warmth.2 Humans, in turn, contrived certain tools, systems, and equipment to help achieve tolerable or ideal ambient temperature inside their shelters.
For instance, radiant heating in the form of steam radiators, radiant in-floor heating, in-wall heating, masonry heaters, and passive solar heating, can warm up surfaces and objects efficiently and produce an even and comfortable heat. Read more facts on Radiant Heating.
Blood homeostasis
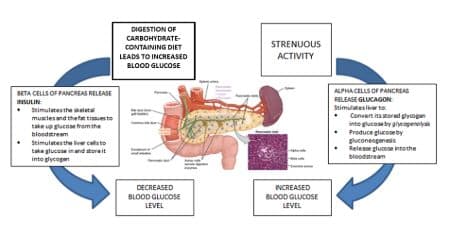
The levels of these components in the blood plasma go through homeostatic regulation. For example, blood sugar level is regulated to set the blood glucose concentration within the tolerable limit. The body maintains homeostasis in this regard largely through the pancreas. The pancreas is a glandular structure made up of two major types of cells: alpha and beta cells.
The alpha cells produce and secrete glucagon whereas the beta cells, insulin. Glucagon and insulin are hormones from the pancreas that regulate glucose concentration in the blood. Insulin, in particular, lowers blood sugar levels by inciting the skeletal muscles, and the fat tissues to take up glucose from the bloodstream.
It also incites the liver cells to take glucose in and store it into glycogen. Conversely, glucagon raises blood sugar levels by stimulating the liver to convert its stored glycogen into glucose by glycogenolysis or produce glucose by gluconeogenesis and release it into the bloodstream.
Thus, when the glucose level is high in the blood circulation (e.g. when consuming a carbohydrate-rich food), the beta cells of the pancreas secrete insulin and inhibit the alpha cells from secreting glucagon. But when glucose level drops (e.g. during an energy-demanding workout), the alpha cells secrete glucagon, and insulin secretion is stopped.
Blood pressure homeostasis
Another instance of a negative feedback loop is the homeostatic regulation of blood pressure. Blood pressure is the force exerted by the circulating blood as it hits the arterial walls. The pressure comes from the heart when it creates a pulsing act. This blood pressure is regulated within the homeostatic range through the cardiovascular system control center. This control center has three distinct activities related to blood pressure regulation3:
- (1) The cardiac center sending nerve impulses to the sympathetic cardiac nerves to increase cardiac output (by increasing heart rate).
- (2) The cardiac center sending nerve impulses to the parasympathetic vagus nerves to decrease cardiac output (by decreasing heart rate).
- (3) The vasomotor center regulating the diameter of blood vessels.
The cardiovascular center receives blood pressure changes information from receptors, e.g. baroreceptors. The baroreceptors are the receptors that are mostly found in the carotid sinus.
They are sensitive to blood pressure changes. For example, when the arterial wall stretches from an increased blood volume, the baroreceptors detect the consequential rise in arterial blood pressure. They send signals to the atrial heart muscle cells to secrete atrial natriuretic peptide (ANP) into the bloodstream.
ANP is a potent vasodilator whose actions include lowering blood pressure. In this regard, its target organ is the kidney which apart from the major function of excreting wastes out of the body as urine it also plays an important role in managing blood volume through the renin-angiotensin-aldosterone system. In particular, ANP stimulates the kidney to stop secreting renin.
Renin is an enzyme that converts angiotensinogen from the liver to angiotensin I. Angiotensin I is converted next by the angiotensin-converting enzyme in the lungs into a potent vasoconstrictor peptide, angiotensin II. The latter causes the target blood vessel to constrict thereby raising peripheral resistance, which may impact blood flow.
An increase in peripheral resistance leads to a rise in blood pressure. Angiotensin II also acts on the adrenal glands by stimulating them to secrete aldosterone. Aldosterone reduces urine output. It does so by entering the principal cells of the distal tubule and the collecting duct of the kidney nephron to bind to the nuclear mineralocorticoid receptor.
This activates the cell to release sodium (Na+) ions via the basolateral Na+/K+ pumps. Three Na+ ions are released out of the cell into the interstitial fluid. Concurrently, 2 K+ ions are taken into the cell from the interstitial fluid. As a result, a concentration gradient causes Na+ ions and water to enter the bloodstream (as for K+ ions, they are secreted from the lumen of the collecting duct into the urine).
The re-absorption of both Na+ ions and water into the blood raises blood volume.
By inhibiting the kidney from secreting renin, its effects and the ensuing events would be inhibited as well. As a result, the blood volume decreases, and the blood pressure drops.
Calcium homeostasis
The chief cells in the parathyroid glands and the parafollicular cells in the thyroid glands are sensory cells that are sensitive to calcium ion (Ca2+) levels. The fall of calcium ions in the plasma incites the chief cells to secrete parathyroid hormone whereas the rise of calcium ions incites the parafollicular cells to secrete calcitonin.4
A drop in Ca2+ level causes the release of parathyroid hormone. The increase of this hormone circulating in the blood incites bone resorption. Also, the hormone causes the excretion of phosphate ions through urine. The excretion of phosphate ions will prevent the latter from binding with Ca2+.
Thereby, unbound Ca2+ can be released into the plasma, correcting the Ca2+ level. Apart from this, the hormone also acts on the kidneys. It stimulates the kidney to release calcitriol into the blood. Calcitriol targets the epithelial cells of the duodenum and jejunum of the small intestine to increase their absorption capacity for calcium from the gut lumen and then release it into the blood. 5
The rise of Ca2+ results in the parafollicular cells secreting calcitonin into the blood. This hormone, in turn, targets the bone cells, stimulating the latter to absorb calcium and convert it into an insoluble form inside the bone, thereby removing excess Ca2+ in the blood.
Potassium homeostasis
The body corrects potassium levels through the action of the adrenal complex. A high concentration of potassium in the plasma leads to the membrane depolarization of the zona glomerulosa in the adrenal cortex.6 This incites the release of aldosterone into the blood. This hormone acts on the kidney.
It stimulates the excretion of excess potassium ions into the urine. It does so via the basolateral sodium/potassium pumps of the tubular epithelial cells. Each of these pumps works by releasing three sodium ions out of the cell and then taking in two potassium ions into the cell.
Because of the resulting ionic concentration gradient, sodium ions are reabsorbed into the blood and then potassium ions are secreted into the lumen of the collecting duct, for their ultimate excretion via the urine.
Osmoregulation
The body fluids of the human body are of two major types: intracellular fluid (fluid inside the cell) and extracellular fluid (fluid outside the cell). Both of these types are made up chiefly of water. The amount of water molecules between these two fluids needs to be regulated and stabilized.
The body does so by osmoregulation. The homeostatic mechanism is initiated by the osmoreceptors in the hypothalamus. These receptors are sensitive to osmotic pressure changes. When these receptors detect hypertonicity (more solute) or hyper-osmolality in the extracellular environment, vasopressin is released into the circulation.
In the case of osmoregulation, vasopressin targets the kidney to exert an antidiuretic response, particularly by promoting water reabsorption, thereby inhibiting further water loss. Apart from the vasopressin release, the hypothalamus also stimulates the thirst center of the brain to increase the urge to drink water.
In the case of hypo-osmolality in the external environment, there is a low plasma vasopressin level. In consequence, water is not reabsorbed from the kidney tubules and therefore excreted into the urine.
Biological Importance of Homeostasis
Homeostasis is important to maintain internal stability and sustain life. Without these homeostatic mechanisms to ensure that the innate variables are kept within the optimal or suitable values, there would be instability in the body.
The system would not be able to function properly and efficiently. In the long run, the individual would get ill, or worse, face death from the failure of the body to rectify rogue variables that impede the system to function as it should.
READ: Physiological Homeostasis and The Human Physiology > Homeostatic Mechanisms and Cellular Communication
NOTE IT!
Fever as Proof that Homeostasis Isn’t Static
While the body aims to maintain a constant internal environment, it could deviate from the norm. For example, the body normally allows the temperature to go relatively higher than the normal range, especially when attempting to combat and inhibit the growth of microbes that have entered the body. Thus, when there is an infection, the body temperature elevates in response to the pyrogens (a group of chemicals triggering fever in the presence of infection). In this case, the hypothalamus is “reset” to a higher temperature, which clinically indicates “fever”.
Fever is a natural immune response of the body. It helps because many pathogens are temperature-sensitive and it promotes a stronger immune response as some immune cells work more effectively at higher temperatures. However, prolonged fever or extremely high temperatures (above 40°C) are serious medical conditions warranting immediate medical attention. At this point, the body’s thermoregulatory mechanism could be overwhelmed and lead to severe adverse effects, such as dehydration and other potential complications. Thus, antipyretic medications are prescribed by physicians to provide relief and lower the body temperature.
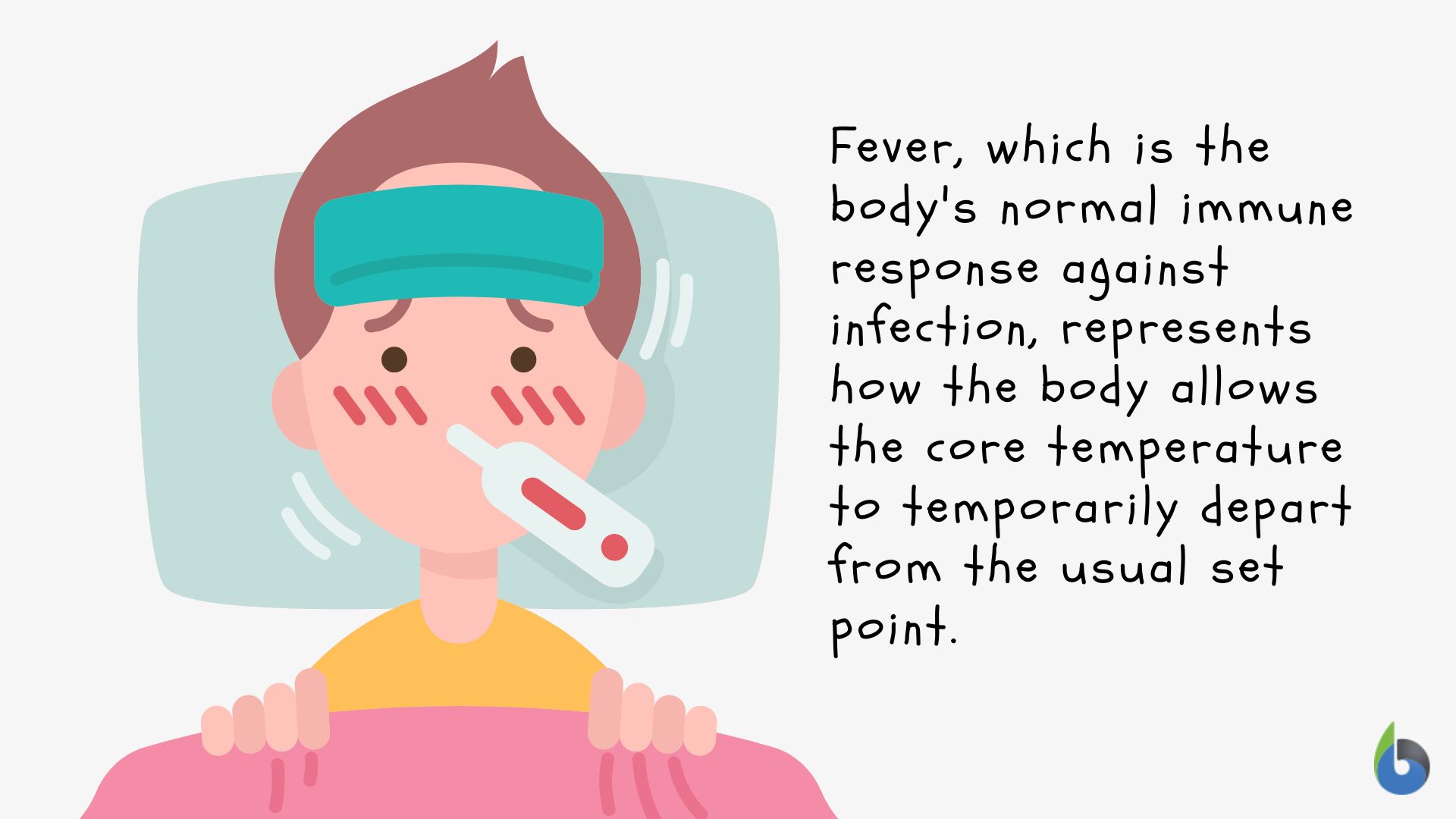
Try to answer the quiz below to check what you have learned so far about homeostasis.
References
- Tansey, Etain A.; Johnson, Christopher D (2015). “Recent advances in thermoregulation”. Advances in Physiology Education. 39 (3): 139–148.
- Campbell, Neil A. (1990). Biology (Second ed.). Redwood City, California: The Benjamin/Cummings Publishing Company.
- Control of Blood Pressure. (2015). Retrieved from Cliffsnotes.com website: https://www.cliffsnotes.com/study-guides/anatomy-and-physiology/the-cardiovascular-system/control-of-blood-pressure
- Brini, M., Ottolini, D., Calì, T., & Carafoli, E. (2013). “Chapter 4. Calcium in Health and Disease”. In Sigel A, Helmut RK (eds.). Interrelations between Essential Metal Ions and Human Diseases. Metal Ions in Life Sciences. 13. Springer. pp. 81–137.
- Stryer, L. (1995). “Vitamin D is derived from cholesterol by the ring-splitting action of light.”. In: Biochemistry (Fourth ed.). New York: W.H. Freeman and Company. p. 707.
- Hu, C., Rusin, C. G., Tan, Z., Guagliardo, N. A., & Barrett, P. Q. (June 2012). “Zona glomerulosa cells of the mouse adrenal cortex are intrinsic electrical oscillators”. J Clin Invest. 122 (6): 2046–2053.
- Palmer, L. G. & Frindt, G. (2000). “Aldosterone and potassium secretion by the cortical collecting duct”. Kidney International. 57 (4): 1324–8.
- Modell, H., Cliff, W., Michael, J., McFarland, J., Wenderoth, M. P., & Wright, A. (2015). A physiologist’s view of homeostasis. Advances in Physiology Education, 39(4), 259–266. https://doi.org/10.1152/advan.00107.2015
- Homeostasis, Steady States, and Equilibria. (2019). Retrieved from Rice.edu website: https://www.ruf.rice.edu/~bioslabs/studies/invertebrates/steadystate.html
- Homeostasis. (2019). Retrieved from Bellarmine.edu website: https://www.bellarmine.edu/faculty/dobbins/microbial/MBernard.htm
- 1.3 Homeostasis Anatomy & Physiology. (2019). Retrieved from Oregonstate.edu website: http://library.open.oregonstate.edu/aandp/chapter/1-5-homeostasis/
- Homeostasis: Positive And Negative Feedback Mechanism. (n.d.). Retrieved from https://www.michigan.gov/documents/explorelabscience/Presentation_on_Homeostasis_560162_7.pdf
- Lecture 21. (2019). Retrieved from Columbia.edu website: http://www.columbia.edu/cu/biology/courses/c2006/lectures08/lect21.08.html
- Animal Organ Systems. (2019). Retrieved from Estrellamountain.edu website: https://www2.estrellamountain.edu/faculty/farabee/biobk/BioBookANIMORGSYS.html
Further Reading
- Radiant Heating Factsheet: Comfortable, Efficient & Healthy. Building Biology Institute. https://buildingbiologyinstitute.org/free-fact-sheets/radiant-heating/
© Biology Online. Content provided and moderated by Biology Online Editors