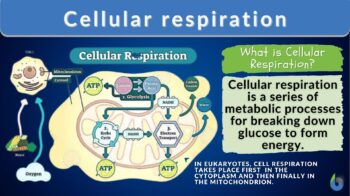
Cellular respiration
n.
ˈsɛljʊlə ɹɛspɪˈɹeɪʃən
Definition: A series of metabolic processes that take place within a cell in which the biochemical energy is harvested from organic substance (e.g. glucose) and then stored in energy-carrying biomolecule (e.g. ATP) for use in energy-requiring activities of the cell
Table of Contents
Cellular Respiration Definition
What is cellular respiration in simple terms? Cellular respiration can be defined simply as a series of metabolic processes that take place within a cell. Biochemical energy is harvested from organic substances (e.g. glucose, a six-carbon molecule) and then stored in energy-carrying biomolecules (e.g. adenosine triphosphate or ATP) for use in the energy-requiring activities of the cell. The main function of cellular respiration is to break down glucose to form energy.
Cellular respiration is a series of metabolic processes that take place within a cell in which the biochemical energy is harvested from an organic substance (e.g. glucose) and then stored in an energy-carrying biomolecule (e.g. ATP) for use in energy-requiring activities of the cell.
Synonyms: cell respiration
Cellular Respiration Overview
Cellular respiration takes place in the cells of all living organisms. What does cellular respiration produce? Cellular respiration produces energy, which is vital because the energy is used to maintain life. The process is carried out by both prokaryotic and eukaryotic cells.
Cellular respiration location
Where does cellular respiration take place? In prokaryotic cells, it is carried out in the cell cytoplasm, in eukaryotic cells it begins in the cytosol and then is carried out in the mitochondria. In eukaryotes, the 4 stages of cellular respiration include glycolysis, transition reaction (pyruvate oxidation), the Krebs cycle (also known as the citric acid cycle), and oxidative phosphorylation through the electron transport chain.
How does cellular respiration work?
Cellular respiration works either in the presence or absence of oxygen. But essentially, the process is called cellular respiration because the cell seems to “respire” in a way that it takes in molecular oxygen (as an electron acceptor) and releases carbon dioxide (as an end product). Hence, the process is described as aerobic.
When the final electron acceptor is not oxygen, it is described as anaerobic. An anaerobic type of respiration is carried out chiefly by anaerobic organisms (e.g. anaerobic bacteria) that use certain molecules as electron acceptors instead of oxygen.
In another anaerobic process, such as fermentation, pyruvate is not metabolized in the same way as an aerobic type of respiration.
The pyruvate molecules produced are not transported into the mitochondria. Rather, they remain in the cytoplasm where they can be turned into a waste product that will be removed from the cell.
Why is cellular respiration important?
The main function of cellular respiration is to synthesize biochemical energy. Cellular respiration is essential to both eukaryotic and prokaryotic cells because this biochemical energy is produced to fuel many metabolic processes, such as biosynthesis, locomotion, and transportation of molecules across membranes.
For the specific products of cellular respiration: jump to the section – What are the Products of Cellular Respiration? For the cellular respiration diagram, see the next section below.
The Location of Cellular Respiration
Cellular respiration takes place in both the cytosol and mitochondria of cells. Glycolysis takes place in the cytosol, whereas pyruvate oxidation, the Krebs cycle, and oxidative phosphorylation occur in the mitochondrion. Figure 1 shows the locations of the main biochemical reactions involved in cellular respiration.
:max_bytes(150000):strip_icc():format(webp)/cellular_respiration-8fcc3f1ad3e54a828dabc02146ce4307.jpg)
The energy produced by the mitochondria is stored as potential energy in molecules called adenosine triphosphate (ATP). The main chemical produced in cellular respiration is ATP. ATP is the standard unit in which the energy released during respiration is stored. The mitochondrion can be recognized as the “powerhouse” of the cell because of its major role in cellular respiration. Mitochondria contain a number of enzymes to aid in this process.
These organelles contain 2 membranes – an outer membrane and an inner membrane. The space in between these membranes is known as the intermembrane space. The outer membrane contains many proteins known as porins and is permeable to molecules and ions (e.g. ATP). The inner membrane contains complexes involved in the electron transport chain stage of cellular respiration which will be described in more detail below.
If cellular respiration takes place in the presence of oxygen, it is known as aerobic respiration. If it takes place in the absence of oxygen, it is known as anaerobic respiration.
Enzyme-catalyzed reactions are responsible for breaking down organic molecules (usually carbohydrates or fats). During these enzyme reactions, a small amount of energy is channeled into molecules of ATP.
ATP is found in every living cell and can relocate energy wherever it is needed. Energy can be released from ATP by its dephosphorylation to adenosine diphosphate (ADP). See Figure 2 for the structure of ATP.
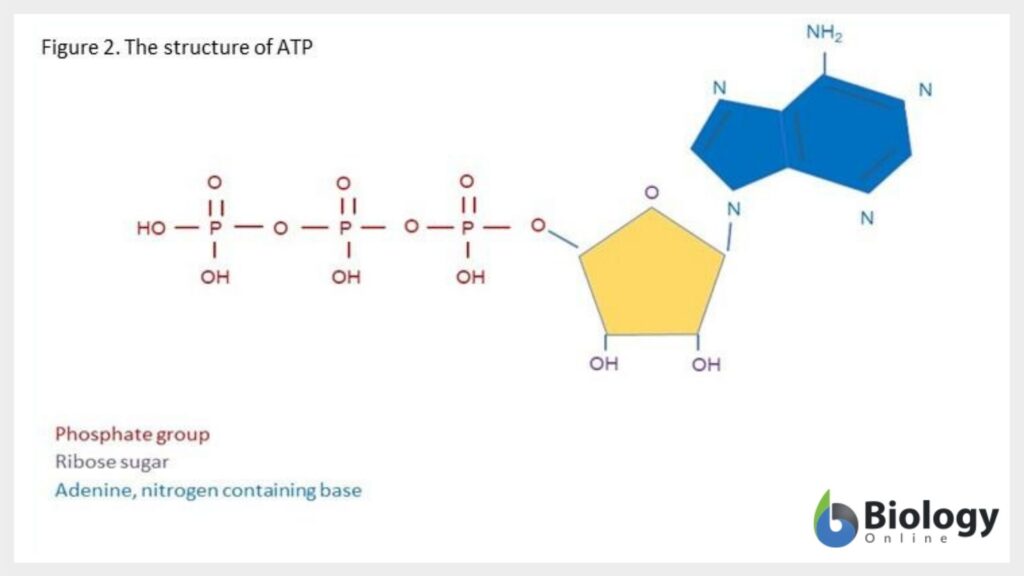
What is the Role of Oxygen in Cellular Respiration?
Oxygen is used in cellular respiration. It is a diatomic molecule (i.e. it is formed of two oxygen molecules joined by a covalent bond) and it is electronegative, meaning it attracts bonding pairs of electrons. As it pulls electrons towards it, it releases energy from the chemical bonds. Potential energy from our food is combined with oxygen and creates products of carbon dioxide (CO2) and water (H2O) which releases energy to form the molecule ATP.
For example, the monosaccharide glucose, (the most basic form of carbohydrate) can be combined with oxygen. The high-energy electrons that are found in the glucose are transferred to the oxygen and potential energy is released. The energy is stored in the form of ATP. This final process of cellular respiration takes place on the inner membrane of the mitochondria. Instead of all the energy being released at once, the electrons go down the electron transport chain.
The energy is released in small pieces and that energy is used to form ATP. See below to understand more about the stages of cellular respiration including the electron transport chain.
Forum Question: How many water molecules are produced by cellular respiration? Featured Answer!
Cellular Respiration Equations
Cellular respiration can be written as chemical equations. An example of the aerobic respiration equation is in Figure 3.
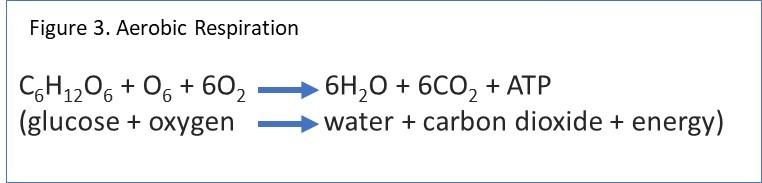
As for chemical equations for anaerobic cellular respiration, see the diagrams below:
- Lactic acid fermentation equation
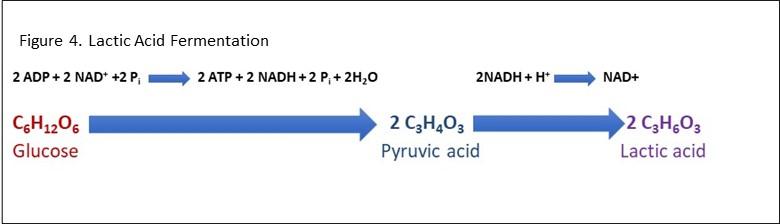
- Alcoholic fermentation equation
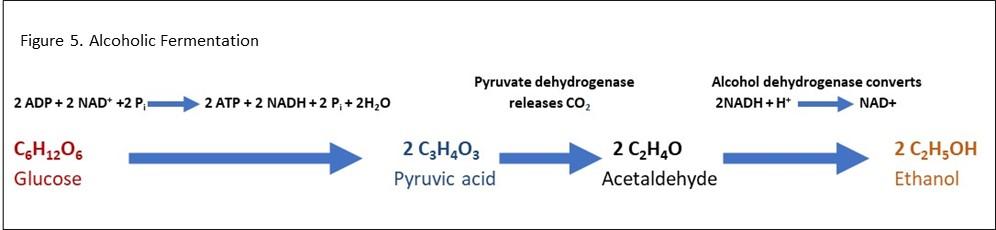
Types of Cellular Respiration
Below are examples of aerobic respiration and anaerobic cellular respiration: lactic acid fermentation and alcoholic fermentation.
Aerobic respiration
Most prokaryotes and eukaryotes use the process of and undergo aerobic respiration. As mentioned above, it is the process of cellular respiration in the presence of oxygen. Water and carbon dioxide are the end products of this reaction along with energy. (See Figure 3)
Lactic Acid Fermentation
In lactic acid fermentation, 6 carbon sugars, such as glucose are converted into energy in the form of ATP. However, during this process, lactate is also released, which in solution becomes lactic acid. See Figure 4 for an example of a lactic acid fermentation equation. It can occur in animal cells (such as muscle cells) as well as some prokaryotes. In humans, the lactic acid build-up in muscles can occur during vigorous exercise when oxygen is not available. The aerobic respiration pathway is switched to the lactic acid fermentation pathway in the mitochondria which although produces ATP; it is not as efficient as aerobic respiration. The lactic acid build-up in muscles can also be painful.
Alcoholic Fermentation
Alcoholic fermentation (also known as ethanol fermentation) is a process that converts sugars into ethyl alcohol and carbon dioxide. It is carried out by yeast and some bacteria. Alcoholic fermentation is used by humans in the process of making alcoholic drinks such as wine and beer. During alcoholic fermentation, sugars are broken down to form pyruvate molecules in a process known as glycolysis. Two molecules of pyruvic acid are generated during the glycolysis of a single glucose molecule. These pyruvic acid molecules are then reduced to two molecules of ethanol and two molecules of carbon dioxide. The pyruvate can be transformed into ethanol under anaerobic conditions where it begins by converting into acetaldehyde, which releases carbon dioxide, and acetaldehyde is converted into ethanol. In alcoholic fermentation, the electron acceptor NAD+ is reduced to form NADH and this exchange of electrons helps to generate ATP. Figure 5 shows an alcoholic fermentation equation.
Methanogenesis
Methanogenesis is a process only carried out by anaerobic bacteria. These bacteria belong to the phylum Euryarchaeota and they include Methanobacteriales, Methanococcales, Methanomicrobiales, Methanopyrales, and Methanosarcinales. Methanogens only occur in oxygen-depleted environments, such as sediments, aquatic environments, and in the intestinal tracts of mammals. There are 3 pathways for methanogenesis:
(1) Acetoclastic Methanogenesis. This process involves activating acetate into acetyl-coenzyme A (acetyl-CoA), from which a methyl group is then transferred into the central methanogenic pathway. Acetoclastic methanogens split acetate in the following way:
CH3COOH (Acetate) –> CO2 (Carbon dioxide) + CH4 (methane)
Acetoclastic methanogenesis is performed by Methanosarcina and Methanosarcinales and is most often found in freshwater sediments. Here, it is thought that acetate contributes to around two-thirds of the total methane formation on Earth on an annual basis.
(2) Methylotrophic Methanogenesis. In methylotrophic methanogenesis, methanol or methylamines serve as the substrate instead of acetate. This process can be observed in marine sediments where methylated substrates can be found. Some acetoclastic methanosarcinales and at least one member of the Methanomicrobiales can also use this second pathway.
(3) Hydrogenotrophic Methanogenesis. Finally, hydrogenotrophic methanogenesis is a process that is used by Methanobacteriales, Methanococcales, Methanomicrobiales, Methanopyrales, and Methanosarcinales (i.e. all five orders). In this reaction, hydrogenotrophic methanogens use hydrogen for the reduction of carbon dioxide, carbon monoxide, or formate according to the following:
4H2 (Hydrogen) + CO2 (Carbon dioxide) –> CH4 (Methane) + 2H2O (Water)
Although methanogenesis is a type of respiration, an ordinary electron transport chain is not used. Methanogens instead rely on several coenzymes, including coenzyme F420, which is involved in the activation of hydrogen, and coenzyme M, which is involved in the terminal reduction of CH3 groups to methane (Figure 6.).
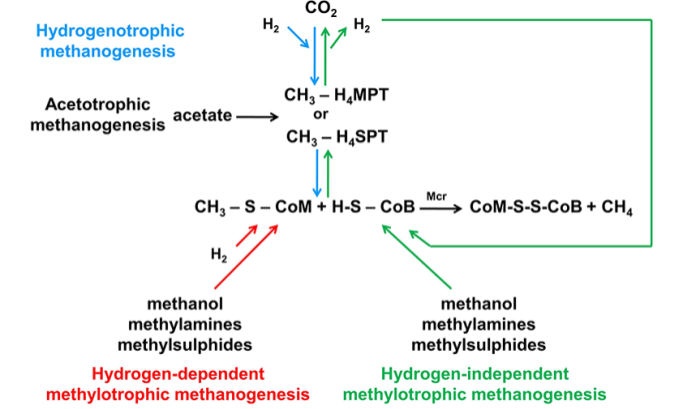
Steps of Cellular Respiration
What are the 4 stages of cellular respiration? There are 4 stages of the cellular respiration process. These are Glycolysis, the transition reaction, the Krebs cycle (also known as the citric acid cycle), and the electron transport chain with chemiosmosis. What does cellular respiration produce? Let’s find out in each of the steps of cellular respiration.
Glycolysis
The literal meaning of glycolysis is ‘splitting sugar’. Glykos comes from the Greek word ‘sweet’ and lysis means ‘to split’. Glycolysis is a series of reactions that extract energy from glucose by splitting it into 2 molecules of pyruvate. Glycolysis is a biochemical pathway that evolved long ago and is found in the majority of organisms. In organisms that perform cellular respiration, glycolysis is the first stage of the process. However, glycolysis doesn’t require oxygen, and many anaerobic organisms also have this pathway.
Before glycolysis begins, glucose must be transported into the cell and phosphorylated. In most organisms, this occurs in the cytosol. The most common type of glycolysis is the Embden–Meyerhof–Parnas (EMP pathway), discovered by Gustav Embden, Otto Meyerhof, and Jakub Karol Parnas. Glycolysis does refer to other pathways, one such pathway described is the Entner–Doudoroff pathway. This article concentrates on the EMP pathway.
The Embden-Meyerhof-Parnas (EMP) pathway of glycolysis
The glycolysis pathway can be separated into two phases:
- The Investment Phase – ATP is consumed.
- The Pay Off Phase – ATP is produced.
Glycolysis takes place in 10 steps. See Figure 7. for a diagrammatic representation of glycolysis.
Step 1.
The enzyme hexokinase phosphorylates glucose using ATP to transfer a phosphate to the glucose molecule to form glucose-6-phosphate. This reaction traps the glucose within the cell.
Step 2.
Glucose-6-phosphate is isomerized into fructose-6-phosphate. This involves the change of an aldose into a ketose. The enzyme phosphoglucose isomerase catalyzes this reaction. A molecule of ATP provides the phosphate group.
Step 3.
Phosphofructokinase (PFK) with magnesium as a cofactor phosphorylates glucose-6-kinase to fructose 1,6-bisphosphate. This enzyme catalyzes the transfer of a phosphoryl group from ATP to fructose-6-phosphate. This reaction yields ADP and fructose 1, 6-bisphosphate.
The importance of phosphofructokinase (PFK)
PFK is a significant enzyme in the regulation of glycolysis. A high ratio of ADP to ATP will lead to the inhibition of PFK and therefore inhibit glycolysis. Adenosine monophosphate (AMP) is a positive regulator of PFK. When ATP levels are low, more ATP is generated by changing ADP molecules to ATP and AMP. When ATP levels are high, PFK is inhibited, thereby slowing down the process of glycolysis. Citric acid is also known to inhibit the action of PFK.
These first 3 stages of glycolysis have used up a total of 2 ATP molecules; hence it is known as the investment phase.
Step 4.
The enzyme aldolase is utilized to split fructose 1, 6-bisphosphate into glyceraldehyde-3-phosphate (GAP) and dihydroxyacetone phosphate (DHAP).
Step 5.
Triosephosphate isomerase reorganizes DHAP into GAP. GAP is the only molecule that continues in the glycolytic pathway. At this point there are two molecules of GAP, the next steps are to fully convert to pyruvate.
Step 6.
Firstly, GAP is oxidized by the coenzyme nicotinamide adenine dinucleotide (NAD) and then it is phosphorylated by the addition of a free phosphate group by the enzyme Glyceraldehyde-3-phosphate dehydrogenase (GAPDH). GAPDH has a form that enables it to hold the GAP in a conformation allowing the NAD molecule to pull hydrogen off it. This results in the conversion of NAD to NADH. The phosphate group then attacks the GAP molecule and releases it from the enzyme to yield 1,3 bisphosphoglycerate, NADH, and a hydrogen atom.
Step 7.
Phosphoglycerate kinase (PGK) with the help of magnesium converts 1,3 bisphosphoglycerate to 3-phosphoglycerate by removing a phosphate group. The phosphate is transferred to a molecule of ADP that yields a molecule of ATP.
Step 8.
Phosphoglycerate mutase rearranges the position of the phosphate group on 3-phosphoglycerate allowing it to become 2-phosphoglycerate.
Step 9.
2-phosphoglycerate is converted to phosphoenolpyruvate (PEP) by the enzyme enolase. Enolase dehydrates 2 phosphoglycerate molecules by removing water.
Step 10.
Finally, pyruvate kinase transfers a phosphate from PEP to ADP yielding ATP and pyruvate.
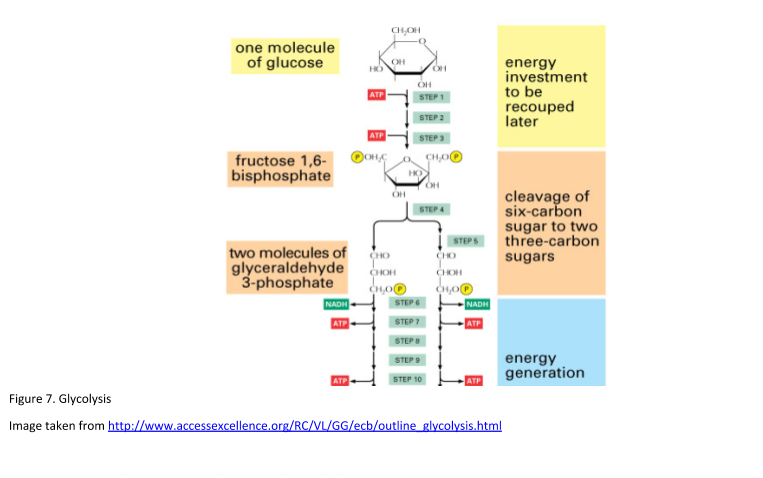
Transition reaction
In aerobic respiration, the transition reaction occurs in the mitochondria. Pyruvate moves out of the cytoplasm and into the mitochondrial matrix. In anaerobic conditions, pyruvate will stay in the cytoplasm and be used in lactic acid fermentation instead. The purpose of the transition reaction is to transfer pyruvate to acetyl CoA producing carbon dioxide and NADH. For every single molecule of glucose, 2 molecules of CO2 and NADH are generated (Figure 8).
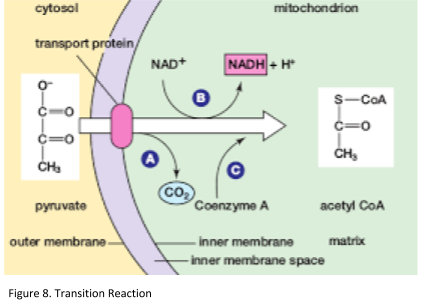
What is the Krebs cycle?
The Krebs cycle, also known as the citric acid cycle, was discovered by Hans Adolf Krebs in 1937. It can be described as a metabolic pathway that generates energy. This process happens in the mitochondrial matrix, where pyruvate has been imported following glycolysis. The final products of the Krebs cycle include 2 molecules of CO2, 3 molecules of NADH, 1 molecule of FADH2, and 1 molecule of GTP. These products are generated per single molecule of pyruvate. The products of the Krebs cycle power the electron transport chain and oxidative phosphorylation. Acetyl CoA enters the Krebs cycle after the transition reaction has taken place (conversion of pyruvate to acetyl CoA). See Figure 9. for the diagrammatic scheme of the Krebs cycle.
There are 8 steps in the Krebs cycle. Below are reviews of some of the principal parts of these steps and the products of the Krebs cycle:
Step 1.
Acetyl CoA joins with oxaloacetate releasing the CoA group and producing citrate, a six-carbon molecule. The enzyme involved in this process is citrate synthase.
Step 2.
Citrate is converted to isocitrate by the enzyme aconitase. This involves the removal and then the addition of water.
Step 3.
Firstly, the secondary OH group of isocitrate is oxidized by the coenzyme NAD+ and a ketone is formed. The ketone is then decarboxylated (i.e. CO2 removed) by isocitrate dehydrogenase leaving behind alpha-ketoglutarate which is a 5-carbon molecule. Isocitrate dehydrogenase is central in regulating the speed of the Krebs cycle citric acid cycle.
Step 4.
Oxidative decarboxylation takes place by alpha-ketoglutarate dehydrogenase. This enzyme catalyzes the conversion of α-ketoglutarate to succinyl-CoA and produces NADH delivering electrons to the respiratory chain.
Step 5.
Succinyl-CoA is converted to succinyl phosphate, and then succinate. Succinate thiokinase (other names include succinate synthase and Succinyl coenzyme A synthetase), converts succinyl-CoA to succinate, and free coenzyme A. It also converts ADP to ATP or guanosine diphosphate (GDP) to guanosine triphosphate (GTP). Firstly, the coenzyme A in the succinyl group is substituted by a hydrogen phosphate ion. Succinyl phosphate then transfers its phosphoric acid residue to guanosine diphosphate (GDP) so that GTP and succinate are produced.
Step 6
Succinate is oxidized to fumarate by succinate dehydrogenase. Flavin adenine dinucleotide (FAD) is the coenzyme bound to succinate dehydrogenase. FADH2 is formed by the removal of 2 hydrogen atoms from succinate. This releases energy that is sufficient to reduce FAD. FADH remains bound to succinate dehydrogenase and transfers electrons directly to the electron transport chain. Succinate dehydrogenase performs this process inside the mitochondrial inner membrane which allows this direct transfer of the electrons.
Step 7
L-malate is formed by the hydration of fumarate. The enzyme involved in this reaction is fumarase.
Step 8
In the final step, L-malate is oxidized to form oxaloacetate by malate dehydrogenase. Another molecule of NAD+ is reduced to NADH during this process.
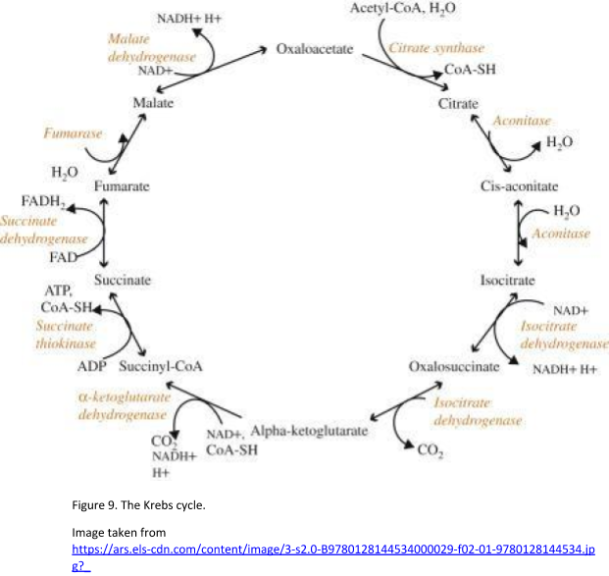
Electron transport chain and chemiosmosis
Where is oxygen used in cellular respiration? It is in the stage involving the electron transport chain. The electron transport chain is the final stage of cellular respiration. It occurs on the inner mitochondrial membrane and consists of several electron carriers.
The purpose of the electron transport chain is to form a gradient of protons that produces ATP. It moves electrons from NADH to FADH2 to molecular oxygen by pumping protons from the mitochondrial matrix to the intermembrane space resulting in the reduction of oxygen to water.
Therefore, the role of oxygen in cellular respiration is the final electron acceptor. It is worth noting that the electron transport chain of prokaryotes may not require oxygen. Other chemicals including sulfate can be used as electron acceptors in the replacement of oxygen.
The complexes involved in the electron transport chain
Four protein complexes are involved in the electron transport chain. Complex I or NADH-ubiquinone oxidoreductase transfers electrons from NADH to Coenzyme Q (ubiquinone). Complex II or succinate dehydrogenase receives FADH2 which is also found in the Krebs cycle. FADH2 transfers its electrons to iron-sulfur proteins within complex II, which then pass the electrons to Coenzyme Q, as for complex I.
These electrons are then shuttled down the remaining complexes and proteins. These include complexes II, III, IV, cytochrome c, and coenzyme Q. They are passed into the inner mitochondrial membrane which slowly releases energy. The electron transport chain uses the decrease in free energy to pump hydrogen ions from the matrix to the intermembrane space in the mitochondrial membranes. This creates an electrochemical gradient for hydrogen ions.
The energy in this gradient is used to generate ATP from ADP and inorganic phosphate (Pi) via the ATP synthase complex. Overall, the end products of the electron transport chain are ATP and water. See Figure 10 for a summary of the electron transport chain.
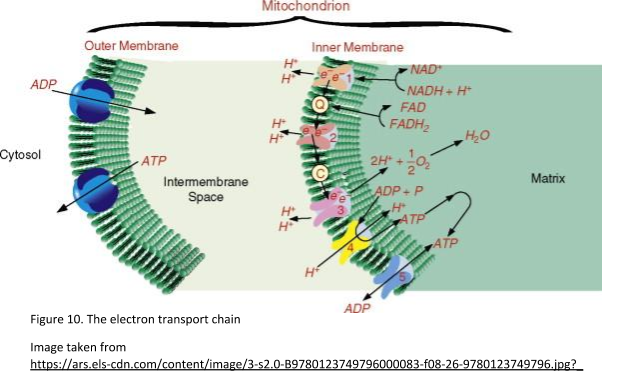
Chemiosmosis
The process described above in the electron transport chain in which a hydrogen ion gradient is formed by the electron transport chain is known as chemiosmosis. After the gradient is established, protons diffuse down the gradient through ATP synthase. The flow of hydrogens catalyzes the pairing of phosphate with ADP, forming ATP (Figure 11). Chemiosmosis was discovered by the British Biochemist, Peter Mitchell. In fact, he was awarded the Nobel Prize for Chemistry in 1978 for his work in this area and ATP synthesis.
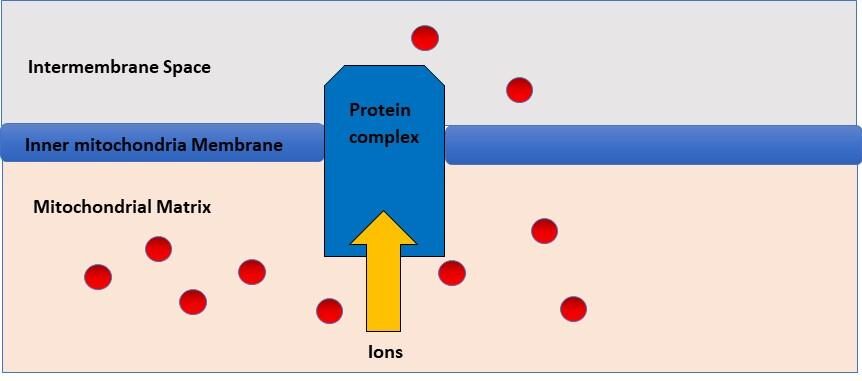
ATP production in cellular respiration
How much ATP is produced in aerobic respiration? What are the products of the electron transport chain? Glycolysis provides 4 molecules of ATP per molecule of glucose; however, 2 are used in the investment phase resulting in a net of 2 ATP molecules. 2 molecules of ATP are produced in the Krebs cycle. Finally, 34 molecules of ATP are produced in the electron transport chain (figure 12).
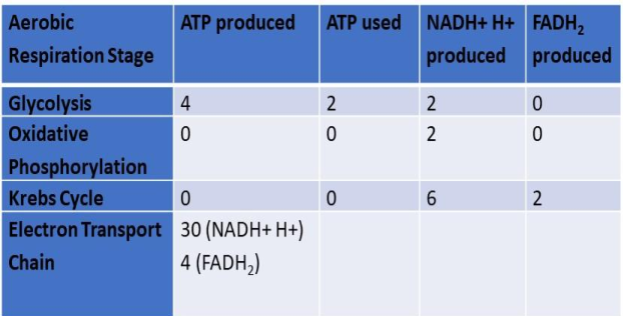
How much ATP is Produced in Fermentation?
Only 2 molecules of ATP are produced in fermentation. This occurs in the glycolysis phase of respiration. Therefore, it is much less efficient than aerobic respiration; it is, however, a much quicker process.
And so essentially, this is how in cellular respiration, energy is converted from glucose to ATP. And by glucose oxidation via the aerobic pathway, more ATPs are relatively produced.
Forum Question: Why is ATP considered as the energy currency? Why not GTP, CTP, or TTP? Featured Answer!
Products of Cellular Respiration
What are the products of cellular respiration? The biochemical processes of cellular respiration can be reviewed to summarise the final products at each stage.
- During glycolysis, the initial reactants are glucose and 2 molecules of ATP, resulting in the end products of pyruvate, ATP, and NADH.
- During the transition reaction, the substrate pyruvate leads to the formation of the products CoA, NADH, and CO2.
- In the Krebs cycle, Acetyl CoA and oxaloacetate lead to the end products oxaloacetate, NADH, ATP, FADH2, and CO2.
- Finally, in the electron transport chain, stage of cellular respiration, NADH, FADH2, ADP, and phosphate are the substrates and the resulting products are NAD, FAD, and ATP.
Cellular Respiration Disorders
Mitochondrial dysfunction can lead to problems during oxidative phosphorylation reactions. This can be due to mutations of either the mitochondrial DNA or the nuclear DNA. These mutations can lead to protein deficiencies. For example, complex I mitochondrial disease is characterized by a shortage of complex I within the inner mitochondrial membrane. This leads to problems with brain function and movement for the individual affected. People with this condition are also prone to having high levels of lactic acid build-up in the blood which can be life-threatening. Complex I mitochondrial disease is the most common mitochondrial disease in children. To date, more than 150 different mitochondrial dysfunction syndromes have been described as related to problems with the oxidative phosphorylation process. Furthermore, there have been over 600 different point mutations in mitochondrial DNA as well as DNA rearrangements that are thought to be involved in various human diseases. There are many different studies ongoing by various research groups around the world looking into the different mutations of mitochondrial genes to give us a better understanding of conditions related to dysfunctional mitochondria.[a][b]
Purpose of Cellular Respiration
What is the purpose of cellular respiration? Different organisms have adapted their biological processes to carry out cellular respiration processes either aerobically or anaerobically dependent on their environmental conditions. The reactions involved in cellular respiration are incredibly complex involving an intricate set of biochemical reactions within the cells of the organisms. All organisms begin with the process of glycolysis in the cell cytoplasm, then either move into the mitochondria in aerobic metabolism to continue with the Krebs cycle and the electron transport chain or stay in the cytoplasm in anaerobic respiration to continue with fermentation (Figure 13). Cellular respiration is the process that enables living organisms to produce energy (ATP) for survival.
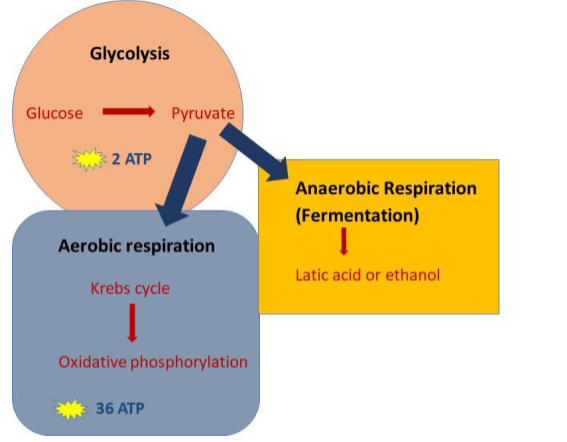
NOTE IT!
“Apart from Glucose, other Biomolecules can enter Cellular Respiration, including the building blocks of DNA and RNA”
While glucose is the most commonly used organic molecule for cellular respiration, other molecules can also be used as fuel.
- Fatty acids: In times of low glucose availability, the body can switch to using alternative energy sources such as fatty acids. Fatty acids can be broken down via beta-oxidation and enter cellular respiration via the citric acid cycle pathway as acetyl-CoA. The approximate ATP yield per fatty acid molecule is ~120 ATP molecules. This is based on the general notion that for every round of beta-oxidation, one molecule of acetyl-CoA is generated. Acetyl-CoA enters the citric acid cycle and produces 3 NADH molecules, 1 FADH2 molecule, and 1 GTP (which later can be converted to ATP).
- Proteins: Proteins can be broken down into amino acids via proteolysis. If the amino acid is used as a fuel, it will first be stripped off of the amino group (deamination) to enter pathways (e.g., citric acid cycle and gluconeogenesis). The breakdown of amino acids generates NADH and FADH2, which can contribute to ATP production through the ETC. The total ATP produced per protein (or per amino acid) will vary depending on the structural composition. On average, some amino acids (e.g., glutamate, aspartate, and arginine) have been estimated to produce about 7 to 10 ATP. Still, this can vary depending on the specific pathway involved and the cellular conditions.
- Nucleic Acids: DNA and RNA can be broken down into nucleotides to be metabolized into intermediates that can enter cellular respiration. Remember that nucleic acids are made up of nucleobases, phosphate groups, and pentose sugars. When degraded, the nucleotide releases pentose sugar (deoxyribose of DNA and ribose of RNA) that may enter different pathways. For instance, ribose-5-phosphate from the breakdown of a ribonucleotide (a building block of RNA) can enter the pentose phosphate pathway, an alternative pathway that eventually leads to the synthesis of glucose-6-phosphate, which can then enter glycolysis. Because of the complexity of the processes prior to the potential use of nucleotides as fuel for cellular respiration, the total ATP cannot be easily derived or estimated.
This flexibility in fuel utilization allows organisms to adapt to different metabolic conditions and energy demands.
Try to answer the quiz below and find out what you have learned so far about cellular respiration.
References
- Bonora, M., Patergnani, S., Rimessi, A., De Marchi, E., Suski, J. M., Bononi, A., Giorgi, C., Marchi, S., Missiroli, S., Poletti, F., Wieckowski, M. R., & Pinton, P. (2012). ATP synthesis and storage. Purinergic signalling, 8(3), 343–357. https://doi.org/10.1007/s11302-012-9305-8
- Britannica Editors. (2020) Sir Hans Adolf Krebs, German British Biochemist. Britannica. https://www.britannica.com/biography/Hans-Krebs
- Cox, S.E. (2013). Energy Metabolism. Encyclopedia of Human Nutrition. (3) 177-185.
- Dautant, A., et al. (2018). ATP synthases diseases of mitochondrial genetic origin. Frontiers in Physiology. (9) 329. https://doi.org/10.3389/fphys.2018.00329
©BiologyOnline.com. Content provided and moderated by BiologyOnline Editors.