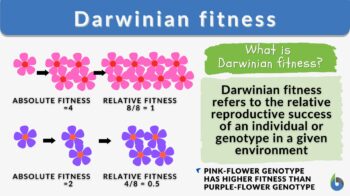
Darwinian fitness
n., plural:
[dɑːˈwɪ.ni.ən fɪtnəs]
Definition: Relative reproductive success of an individual or genotype in a given environment
Table of Contents
Darwinian Fitness Definition
Darwinian fitness refers to the measure of an individual organism’s or genotype‘s reproductive success or the ability of an organism to pass on the genes to the next generation in a given environment. The name is credited to Charles Darwin, the British biologist who is widely known for his theory of natural selection. Individuals (or genotypes) with higher fitness are more likely to survive and reproduce. Consequently, they will also have a higher likelihood to pass on the advantageous traits to their offspring, resulting in the spread of those traits in a population over time.
Before we define Darwinian fitness in the context of evolutionary biology and theoretical population biology, let us have an overview of the meaning of fitness in natural selection and evolution below.
- What does fitness mean in biology?
Fitness, in biology, is not just about physical strength as it would have been defined in a general, broader context. Instead, it is about the overall capacity of an organism to pass on its DNA to its progeny. It is a relative measure, meaning it depends on the interaction between an organism’s traits and the environment in which it lives. It would additionally rely on essential traits, such as resistance to diseases, behavioral attributes for efficient mating and parental care, and greater adaptability to varying ecological factors (environmental conditions) leading to successful reproduction.
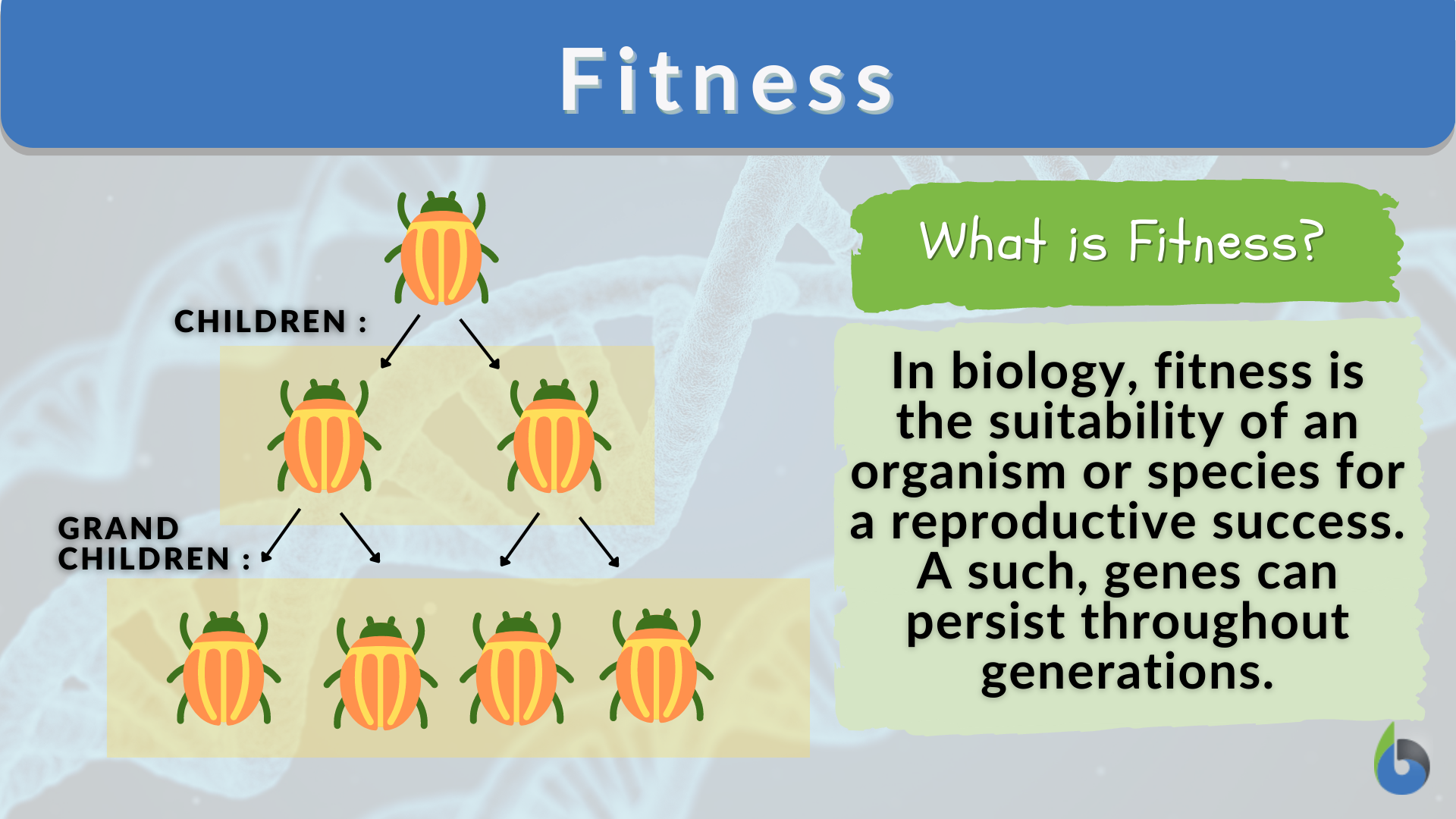
- What is evolutionary fitness? Is it the same as Darwinian fitness?
Evolutionary fitness and Darwinian fitness are at times used interchangeably. In fact, there are other terms that appear to have a similar context. These are reproductive fitness, biological fitness, and relative fitness. They all refer to the same concept of measuring the ability to survive and reproduce successfully in a certain environment.
- In simple terms, what is meant by fitness in Darwinian evolution?
In simple terms, Darwinian fitness (or evolutionary fitness) reflects how well a particular type of organism (or genotype) can survive and take over in a competition for resources, including mates. The key idea behind it is that organisms within a population vary in their traits, and some of these variations provide advantages in the context of their environment.
- How is Darwinian fitness calculated?
The calculation of Darwinian fitness typically depends on the mode of reproduction (whether asexual or sexual):
» Asexual: Darwinian fitness can be calculated in a more straightforward manner by counting the number of offspring produced by fitness. Example: If an asexual individual A produces 10 offspring, the fitness of individual A would be 10.
» Sexual: Calculation of Darwinian fitness is relatively complex. It entails controlled breeding conditions and comparing the reproductive success of different genotypes within a population. The number of offspring produced by each cross is counted and then the relative fitness of each genotype is obtained. Another approach is to calculate fitness based on the frequency of a genotype in subsequent generations. By tracking the changes in genotype frequencies over generations, the fitness of the different genotypes can be inferred.
Watch this vid about Darwinian fitness:
Biology definition:
Darwinian fitness is a fundamental concept in evolutionary biology and is based on Charles Darwin’s theory of natural selection. Darwinian fitness is a measure of the relative reproductive success of an organism in passing its genes to the next generation’s gene pool.
Darwinian fitness differs from “physical fitness”, which is more associated with health, muscle tissues, oxygen, and physical effort. Darwinian fitness is more concerned with reproductive success, i.e., how successful an organism has been at passing on its genes. The more likely that an individual is able to survive and live longer to reproduce, the higher the fitness of that individual. Thus, it may indicate the relative measure of the reproductive success of an organism in passing its genes to the next generation. It denotes the relative ability of an individual (or population) to survive, reproduce and propagate genes in an environment. Thus, when one says an organism is biologically fit, it could mean that the organism is adapted and suitable to its environment based on its relative reproductive success with respect to its population.
Etymology: derived from the surname of Charles Darwin, the British biologist known for his theory of evolution through natural selection.
Synonym: Reproductive fitness; Biological fitness; Evolutionary fitness; Relative fitness
Fitness Is A Propensity
How can one say if an organism is ‘fitter’ than the other? How can we tell that a trait is advantageous or effective at improving ‘fitness’?
The term “propensity” generally refers to a tendency, a predisposition, or a natural inclination. It speaks about ‘probability’. Thus, in this context of evolutionary biology, the propensity interpretation of fitness refers to the natural tendency of an organism to survive, reproduce, and pass on its genes in a specific environment. Just like how some organisms have an instinctive tendency to vie for limited resources, some would also have the innate tendency to have offspring more than others.
The concept of “fitness as a propensity” (PIF) refers to the idea that an organism’s fitness is not a fixed value; rather, it is a probabilistic measure that influences its ability to survive, reproduce, and pass on its genes to the next generation. Fitness is not deterministic but involves probabilities, allowing for variations and uncertainties in reproductive outcomes.
The concept of fitness is a complex and multifaceted topic. PIF is one of the widely discussed and debated. There are other perspectives attempting to interpret fitness. Statistical view, for instance, is a perspective wherein fitness is treated as a statistical property. It makes use of mathematical models and statistical methods to understand the distribution of traits and genes in populations in relation to the population’s reproductive success. Inclusive fitness and kin selection, in turn, is where the role of genetic relatedness in determining fitness is emphasized.
Models Of Fitness: Asexuals and Sexuals
Fitness in asexual organisms is a case in point wherein fitness is illustrated through reproductive models sans the complexity of sex and recombination. That way fitness can be measured and attributed directly to genotypes. Conversely, fitness in sexual organisms is more complex. It would have to consider the reproductive successes of different genotypes within a population.
There are two ways through which fitness can be measured: absolute fitness (W) and relative fitness (w). Both of them can tell how well a specific genotype or genetic trait is performing in a population. They differ in their approach and interpretation.
Absolute Fitness
Absolute fitness refers to the overall reproductive success of an individual asexual organism. It quantifies the proportional change in the abundance of a genotype over one generation solely due to natural selection.
Relative fitness
Relative fitness is a measure of the comparison of the absolute fitness of one individual or genotype to the absolute fitness of other individuals or genotypes in the population.
To define relative fitness (w): Absolute fitness of a genotype / Absolute fitness of a reference genotype of the same population (often, the most successful or fittest in a population)
Note: Relative fitness that is equal to 1 indicates reference genotype
In summary, absolute fitness refers to the raw (exact) number of offspring an organism has over one generation whereas relative fitness is the fitness ratio.
Sample Exercise 1 (Asexual population)
See Table 1 below. Based on the relative fitness (w) of each genotype, which genotype would be considered the most “fit”?
Table 1: Relative fitness of each genotype | |||
---|---|---|---|
AA | Aa | aa | |
Absolute Fitness | 20 | 10 | 5 |
Relative fitness | 20/20 = 1.0 | 10/20 = 0.50 | 5/20 = 0.25 |
Answer:
If reproductive rates are the same for the three genotypes, then relative fitnesses can be derived by dividing the absolute fitnesses by the highest absolute fitness value, which in this example is 20. So, if the respective relative fitnesses are as follows: 1.0 for AA, 0.50 for Aa, and 0.25 for aa, then, AA is the most fit or most successful among the three genotypes in that given environment at that particular time.
Change in genotype frequencies due to selection
Genotypes with higher fitness, i.e., those that produce more offspring, are more likely to become more prevalent in the population. Conversely, those with lower fitness may decrease in frequency or disappear. The process of selection is, therefore, driven by the differential reproductive success of genotypes and their ability to adapt to the prevailing environmental conditions.
To calculate the change in genotype frequencies due to selection, one can make use of relative fitness:
where p represents the frequency of one allele and q represents the frequency of another allele in the population.
Sample Exercise 2 (Sexual population)
Consider these offspring data on fly population. If A1A1 individual cells produce, on average, 23 offspring, A1A2 individual cells produce, on average, 26 offspring, and A2A2 individual cells produce, on average, 28 offspring each generation.
Question: Using relative fitness and assuming the Hardy-Weinberg equilibrium, how will the genotype frequencies in the population change or evolve?
Answer:
1. Let’s calculate the relative fitness (w) of each genotype using A2A2 as the reference genotype (highest)
Table 2: Relative fitness of each genotype | |||
---|---|---|---|
A1A1 | A1A2 | A2A2 | |
The average number of offspring | 23 | 26 | 28 |
Relative fitness (w) | 23/28 = 0.8214 | 26/28 = 0.9286 | 28/28 = 1.0000 |
2. We can now use the values to calculate the change in genotype (or allele) frequencies in one generation through the process of selection.
In the absence of evolutionary forces (mutation, selection, migration, and genetic drift), genotype frequencies in a population are assumed to remain constant from one generation to the next and will then be calculated using the Hardy-Weinberg equation. Since we have three genotypes (A1A1, A1A2, and A2A2) in the population, the sum of their frequencies will be represented in this formula equal to 1: p2 + 2pq + q2 = 1.
where:
p = frequency of the A1 allele
q = frequency of the A2 allele
If the initial genotype frequencies are at Hardy-Weinberg equilibrium, we will assume the frequencies as follows:
p2= Frequency of A1A1 individuals = 0.25 (25%)
2pq = Frequency of A1A2 individuals = 0.50 (50%)
q2 = Frequency of A2A2 individuals = 0.25 (25%)
Updating the genotype frequencies based on the relative fitness values, we get:
(1) Updated frequency of A1A1 individuals = p2 * wA1A1 ≈ 0.25 * 0.8214 ≈ 0.2054
(2) Updated frequency of A1A2 individuals = 2pq * wA1A2 ≈ 0.50 * 0.9286 ≈ 0.4643
(3) Updated frequency of A2A2 individuals = q2 * wA2A2 ≈ 0.25 * 1.0000 ≈ 0.2500
The sum of the updated frequencies should be equal to 1. To normalize, divide each updated frequency by the sum of all updated frequencies:
(1) Normalized frequency of A1A1 individuals
≈ (p2 * 0.8214) / [(p2 * 0.8214) + (2pq * 0.9286) + (q2 * 1.0000)]
≈ 0.2054 / (0.2054 + 0.4643 + 0.2500) 0.9197
≈ 0.223 (22.3%)
(2) Normalized frequency of A1A2 individuals
≈ (2pq * 0.9286) / [(p2 * 0.8214) + (2pq * 0.9286) + (q2 * 1.0000)]
≈ 0.4643 / (0.2054 + 0.4643 + 0.2500)
≈ 0.505 (50.5%)
(3) Normalized frequency of A2A2 individuals
≈ (q2 * 1.0000) / [(p2 * 0.8214) + (2pq * 0.9286) + (q2 * 1.0000)]
≈ 0.2500 / (0.2054 + 0.4643 + 0.2500)
≈ 0.272 (27.2%)
Interpretation:
After one generation of selection based on the given values and assuming the initial genotype frequencies at Hardy-Weinberg equilibrium and in the absence of evolutionary forces (e.g., mutation, selection, migration, and genetic drift), the genotype frequencies of the fly population changed. The genotype A1A2 eventually became more prevalent in the population as it increases in frequency from its initial value whereas the A1A1 and A2A2 genotypes become less common.
History
The term “fitness” as used in biology is attributed to the renowned British naturalist Charles Darwin, who laid the groundwork for the theory of evolution through natural selection that got published in 1859. Darwin proposed that species change over time through the process of natural selection. Individuals in a population will show natural variation in their traits and some of these traits will become more “fit” or advantageous to their environment than others. Advantageous in the sense that such traits augmented the propensity of the individuals to survive, reproduce, and pass on these traits to their offspring. This led to the gradual evolution of species through generations.
The phrase “survival of the fitness” though was not explicitly used in Darwin’s writings. It was the British sociologist Herbert Spencer that coined the phrase “survival of the fittest” in his 1864 work, “Principles of Biology”. The term “fitness” in the context of evolutionary biology was later popularized and expanded upon by subsequent scientists and evolutionary biologists in the 20th century, such as Ronald A. Fisher, J.B.S. Haldane, and Sewall Wright. J.B.S. Haldane, for instance, was the first to formally conceptualize fitness as a quantitative measure of reproductive success in populations.
Genetic Load
The genetic load refers to the impact of harmful or deleterious genetic mutations within a population. As harmful mutations accumulate over time, the genetic load increases. This means there is an increased tendency that may reduce overall fitness and adaptability. The genetic load can be measured through the fitness of a certain reference genotype (e.g., a theoretical optimum or the maximum fitness observed in the population) represented by and the fitness of an average genotype in a population represented by
.
Take note that the concept of maximum fitness is theoretical and may not be achievable in natural populations. It is just an assumption that an organism would have all the possible advantageous traits for its environment leading to the highest reproductive output and success. In reality, the reproductive output will depend on various external factors, like competition and environmental changes. Nevertheless, maximum fitness is a useful concept to gauge the potential of an organism to adapt and successfully reproduce in a particular environment, and thus, measure the likelihood of its species to gradually evolve over time.
Frequently Asked Questions On Darwinian Fitness
What is the role of Darwinian fitness in evolution?
Darwinian fitness is crucial in evolution. It provides a means through which species change and adapt to their environment through time. Individuals or genotypes with higher fitness contribute more of their genetic material to the gene pool of the next generation, leading to the spread of beneficial traits and the gradual evolution of populations. Through natural selection, favorable traits that have become prevalent in a population could drive the adaptation and evolution of species over generations.
As natural selection acts upon this variation in fitness, traits that are “advantageous” could increase an organism’s likelihood of surviving, reproducing, and having offspring that could, in turn, pass on those traits to their offspring, and then, their offspring’s offspring. These traits would therefore become more common in a population over time This could lead to a population that is more adapted to its environment as the advantageous traits become prevalent.
How is Darwinian fitness measured?
In evolutionary terms, an organism’s fitness is measured by its ability to pass on its genes to subsequent generations in a specific environment. Darwinian fitness could be understood through quantification, which is often done through computational and numerical methods allowing for a broad predictive measure of fitness, considering resource constraints and other evolutionary parameters. Such methods aid in assessing the relative reproductive success of individuals or genotypes in a population. These led to models aimed at measuring Darwinian fitness and predicting evolutionary outcomes.
Now, recall that the term Darwinian fitness refers to the ability of individuals to survive, reproduce, and pass on their genes. This ability entails competition for resources in their environment. The outcome of such a competition is said to be an event that can be predicted in a given environment. That’s what classical models are used for. Models of this dynamical process claim that the outcome of the competition is a deterministic event that depends on the population growth rate. Such a measurable factor related to how fast the population grows pertains to the Malthusian parameter. (Demetrius & Ziehe, 2007)
Recent studies contend that such precept is only applicable to infinite population sizes.
For a finite or small population size, the outcome of the competition is rather “a stochastic process—contingent on resource constraints“. (Demetrius & Ziehe, 2007) It means the outcome of the competition is not so predictable. It is more of a stochastic process that depends on how quickly the population goes back to its normal state after some random changes in birth and death rates. This return rate, which measures population stability, is recognized by a demographic parameter “evolutionary entropy“, such as that measured in a study by Demetrius & Ziehe (2007). Accordingly, their computational analysis rejects the Malthusian model and supports the entropic principle as the appropriate measure of Darwinian fitness and evolutionary trajectory.
Apart from absolute fitness and relative fitness, are there any other measures of evolutionary fitness?
Other possible measures of evolutionary fitness are as follows:
- Survival fitness, an organism’s ability to survive to reproductive age and then contribute its genes to the next generation is assessed
- Reproductive success (or fertility fitness), an organism’s ability to reproduce viable offspring and their subsequent survival is assessed
- Ecological fitness, an organism’s adaptation to its ecological niche along with its ability to obtain resources, avoid predators, and compete for mates (sexual fitness) is assessed
- Lifetime reproductive success, the total number of viable offspring an individual produces over its entire lifespan is measured
- Inclusive fitness is when an organism’s direct reproductive success together with the reproductive success of its close relatives are taken into account
- Malthusian parameter, where the rate of population growth based on the organism’s reproductive output is measured. It entails taking the natural logarithm of the population size at a particular time and then dividing it by the generation time
- Selection coefficient, used in population genetics to quantify the strength of natural selection acting on specific genetic traits.
Take the Darwinian Fitness – Biology Quiz!
References
- Frank, S. A. (1995). George Price’s Contributions to Evolutionary Genetics. Journal of Theoretical Biology, 175(3), 373-388.
- Houle, D. (1992). Comparing Evolved Response to Directional Selection on Laboratory and Wild Populations. Genetica, 86(3), 337-347.
- Endler, J. A. (1986). Natural Selection in the Wild. Princeton University Press.
- Demetrius, L., & Ziehe, M. (2007). Darwinian fitness. 72(3), 323–345. https://doi.org/10.1016/j.tpb.2007.05.004
- Rosenberg, A., & Bouchard, F. (2015). Fitness (Stanford Encyclopedia of Philosophy). Stanford.edu. https://plato.stanford.edu/entries/fitness/#ClaProFit
- Suárez, M. (2022). The Complex Nexus of Evolutionary Fitness. 12(1). https://doi.org/10.1007/s13194-021-00434-w
- | Darwin Correspondence Project. (2023). Darwin Correspondence Project. https://www.darwinproject.ac.uk/letter/DCP-LETT-5140.xml#back-mark-5140.f5
©BiologyOnline.com. Content provided and moderated by Biology Online Editors.